WDM – Wavelength Division Multiplexing
A distinctive operational feature of an (WDM wavelength division multiplexing) optical fiber is that there is a wide spectral region in which optical signals can be transmitted efficiently. For full- spectrum fibers this region includes the O-band through the L-band, which ranges from about 1260 to 1675 nm.
Since the light sources used in high-capacity optical fiber communication systems emit in a narrow wavelength band of less than 1 nm, then many different independent optical channels can be used simultaneously in different segments of this wavelength range.
The technology of combining a number of such independent information-carrying wavelengths onto the same fiber is known as wavelength division multiplexing or WDM1-10.
The post addresses the operating principles of WDM, examines the functions of a generic WDM link, and discusses the internationally standardized spectral grids for two different wavelength multiplexing schemes.
Sections 10.2 through 10.6 describe various categories of passive optical components that are needed to combine wavelengths at the transmitting end and separate them back into individual channels at the destination.
An important factor in deploying these components in a WDM system is to ensure that optical signal power from one channel does not drift into the spectral territory occupied by adjacent channels10.
The design of complex wavelength division multiplexed links requires choosing many optical sources with narrow spectral emission bands. The most straightforward method is to select a series of individual lasers, each of which emits at a specific wavelength.
This selection process is adequate for a small number of wavelength channels, but can be cumbersome for links carrying many wavelengths. To simplify this process, a variety of wavelength- tunable components and schemes have been investigated concerning the administration of channels in a WDM transmission system. Among these are tunable light sources, which Sec. 10.7 addresses, and tunable optical signal conditioning and receiving devices, which 10.8 describes. Chapters 11 and 13 define additional active components used for signal amplification and for switching and routing of individual wavelengths.
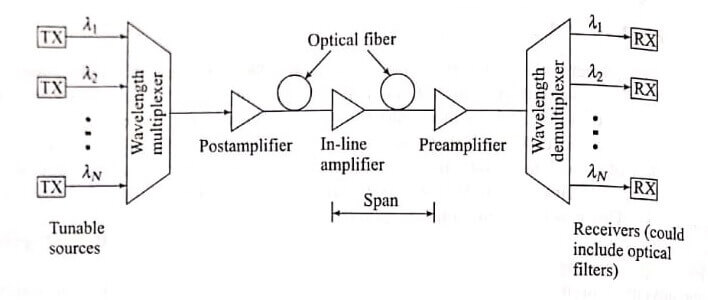
Applications of WDM techniques are found in all levels of communication links including long-distance terrestrial and undersea transmission systems, metro networks, and fiber-to-the-premises (FTTP) networks. Befor post shows how WDM methodologies apply to several categories of communication networks.
Overview of WDM
The original optical fiber links that were deployed around 1980 consisted of simple point-to-point connections. These links contained a single fiber with one light source at the transmitting end and one photodetector at the receiving ent. In these early systems, signals from different light sources used separate and uniquely assigned optical fibers.
Since the spectral width of a typical laser source occupies only a narrow slice of optical bandwidth, these simplex systems greatly underutilize the large bandwidth capacity of a fiber. The first use of WDM was to upgrade the capacity of installed point-to-point transmission links. This was achieved with wavelengths that were separated from several tens up to 200 nanometers in order not to impose strict wavelength-tolerance requirements on the different laser sources and the receiving wavelength-separating components at the receiving end.
With the advent of high quality light sources with extremely narrow spectral emission widths (less than 1 nm), many independent wavelength channels spaced less than a nanometer apart could be placed on the same fiber. For example, the modulated output of a DFB laser has a frequency spectrum of 10 to 50 MHz, which is equivalent to a spectral emission width of less than 10-3nm.
With such light sources the use of WDM allows a dramatic increase in the capacity of an optical fiber compared to the original simple point to point link that carried only a single wavelength. For example, if each wavelength supports an independent transmission rate of 10 Gb/s, then each additional channel provides the fiber with significantly more capacity.
Another advantage of WDM is that the various optical channels can support different transmission formats.
Thus, by using separate wavelengths, differently formatted signals at any data rate can be sent simultaneously and independently over the same fiber without the need for a common signal structure.
Operational Principles of WDM
A operational of WDM is that the discrete wavelengths form an orthogonal set of carriers which can be separated, routed and switched without interfering with each other.
This isolation between channels hold as long as the total optical power intensity is kept sufficiently low to prevent nonlinear effects such as stimulated Brillouin scattering and four-wave mixing processes from degrading the link performance (see next post).
The implementation of sophisticated WDM networks requires a variety of passive and active devices to combine, distribute, isolate, and amplify optical power at different wavelengths. Passive devices require no external control for their operation, so they are somewhat limited in their application flexibility. These components are mainly used to split and combine or tap off optical signals.
The wavelength-dependent performance of active devices can be controlled electronically or optically, thereby providing a large degree of network flexibility.
Active WDM Wavelength Division Multiplexing Concepts and Components components include tunable optical filters, tunable sources, and optical amplifiers.
Image shows the implementation of passive and active components in a typical WDM link containing various types of optical amplifiers.
At the transmitting end there are several independently modulated light sources, each emitting signals at a unique wavelength. Here a multiplexer is needed to combine these optical outputs into continues spectrum or signals and couple them onto a single fiber.
At the receiving end a demultiplexer is required to separate the optical signals into appropriate detection channels for signal processing.
As shows the image, there are many independent operating regions across the spectrum ranging from the O-band through the L-band in which narrow linewidth optical sources can be used simultaneously. We can view these regions either in terms of spectral width (the wavelength band occupied by the light Since WDM is essentially frequency division multiplexing at optical carrier frequencies, the WDM standards developed by the International Telecommunication Union (ITU) specify channel spacing in terms of frequency11-13.
A key reason for selecting a fixed frequency spacing, rather than a constant wavelength spacing, is that when locking a laser to a particular operating mode, it is the frequency of the laser that is fixed.
Recommendation G.692 was the first ITU-T specification for WDM11. This document specifies selecting the channels form a grid of frequencies referenced to 193.100 THz (1552.524 nm) and spacing them 100 GHz.
- See More : Electromagnetic waves
- See More : Radio over fiber links
- See More : Penalty power in optical communication